O2 A-Band Photoacoustic Spectroscopy
The goal of the oxygen A-band project is to provide high accuracy spectroscopic parameters based on fundamental collisional physics for the Orbiting Carbon Observatory-2 (OCO-2), a NASA satellite mission measuring global sources and sinks of carbon dioxide. Atmospheric carbon dioxide levels have been increasing exponentially since the Industrial Revolution leading to elevated surface temperatures due to the greenhouse effect. However, on average only about half of the emitted CO2 accumulates in the atmosphere each year. Understanding the sources and sinks of carbon dioxide is critical for understanding how the Earth will respond to future increases in CO2.
OCO-2 is equipped with three grating spectrometers that use reflected sunlight to measure two carbon dioxide bands (1.61 µm and 2.06 µm) as well the oxygen A-band (762 nm). Since oxygen is uniformly distributed throughout the atmosphere, the oxygen A-band measurements are used for determining air mass, solar pathlength and surface pressure, so errors in oxygen spectroscopy propagate through to the retrieved column CO2 values. The goal of OCO-2 is to retrieve the dry column CO2 mole fraction with 0.25% accuracy; currently, the largest spectroscopic uncertainties are line mixing (LM) and collision-induced absorption (CIA) in the A-band. Line mixing is the collisional transfer of energy between closely spaced energy levels and results in the transfer of intensity between absorption lines. Collision-induced absorption arises from the short-lived dipoles induced during a collision which can allow for the absorption of radiation. These collisional effects impact the spectra in the wings and baseline and become more prominent at elevated pressures.
(A) Oxygen A-band at 762 nm, (B) Weak CO2 band at 1.61 µm, (C) Strong CO2 band at 2.06 µm (Figure from Eldering et al., Science, 358 (6360), 2017)
To improve these spectroscopic uncertainties in line mixing and collision-induced absorption, the Okumura group uses photoacoustic spectroscopy. The large dynamic range and zero-background advantages of this technique allow us to to measure unsaturated, high SNR spectra over a wide pressure range to quantify LM and CIA. The spectrometer is capable of measuring both the P and R-branches of the A-band up to J'=28 with a signal-to-noise ratio of 30,000 for pressures of 50-4,000 Torr. A temperature control system has also been implemented to allow for measurements over the range of atmospherically relevant temperatures. This project involves instrument development, data collection, and spectral fitting as well as collaborations with JPL and NIST.
(Figure from E.M. Lunny, High-Resolution Photoacoustic Spectroscopy of the Oxygen A-Band, 2020)
References:
Eldering et al., The Orbiting Carbon Observatory-2: first 18 months of science data products, Science, 358 (6360), 2017: https://amt.copernicus.org/articles/10/549/2017/
E.M. Lunny, High-Resolution Photoacoustic Spectroscopy of the Oxygen A-Band, 2020: https://resolver.caltech.edu/CaltechTHESIS:06082020-132244698
Drouin et al., Multispectrum analysis of the oxygen A-band, J Quant Spectrosc Radiat Transf., 186 (118-138), 2017: https://www.ncbi.nlm.nih.gov/pmc/articles/PMC5103325/
Spectroscopy with Interband Cascade Lasers
Optical frequency combs have unique properties making them ideal for multiplexed detection of trace molecules. They are composed of many discrete modes at regular intervals, providing broadband coverage while keeping the benefits of a continuous wave laser. We are collaborating with Dr. Mahmood Bagheri at JPL, who has developed frequency combs based on interband cascade lasers, which are chip-scale devices which operate in the CH stretching region. We are developing new cavity-enhanced direct frequency comb methods using interband cascade lasers for investigating trace species in radical reactions.
- L.A. Sterczewski et.al. Cavity-Enhanced Vernier Spectroscopy with a Chip-Scale Mid-Infrared Frequency Comb, ACS Photonics, 2022 https://doi.org/10.1021/acsphotonics.1c01849
Planetary and Astrochemistry
The evolution of the chemical composition of planetary atmospheres is an active area of research. There is abundant evidence that Mars used to have flowing water; however, present-day Mars has no liquid water. One key to understanding the mechanism of water loss is the atmospheric D/H ratio, which is 6x enriched in deuterium relative to Earth's standard mean ocean water. This enrichment is consistent with preferential escape of lighter H atoms over D atoms from the top of the Martian atmosphere.
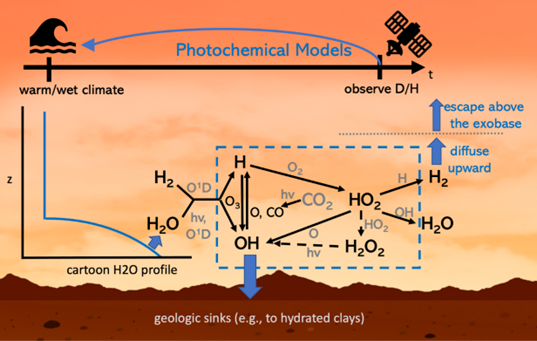
The process of Martian water loss is outlined above. Water is photolyzed, chemically transformed to long-lived H2, then transported to the top of the atmosphere, after which it can escape. While the chemistry underpinning the transformation of water to H2 is thought to be well understood, the effect of isotopic substitution on reaction rates is poorly characterized, or in many cases, entirely unstudied!
In the Okumura group, we are working on characterizing the kinetic isotope effect (KIE) of various HOx reactions in the Martian atmosphere, using both quantum chemical/in silico methods and direct experimental measurement.
Future interests include study of reactions relevant to other planetary atmospheres and the application of spectroscopic techniques for the search for life in our solar system.
Related Projects
- Kinetic Isotope Effect
- HOx chemistry of the Martian atmosphere